DBP:Atrial Fibrillation
ATRIAL FIBRILLATION
PI: Rob MacLeod, University of Utah
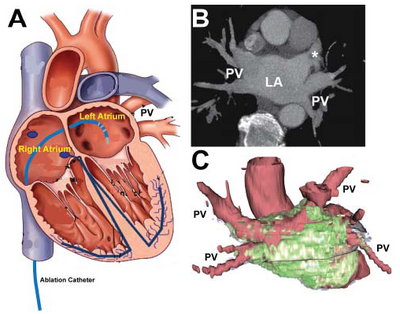
Approximately 0.5% of patients have AF in the 50 to 59 year age group, and up to 9% have AF in the 80 to 89 year age group. Moreover, the prevalence is increasing [1]. AF is associated with increased morbidity (i.e., stroke) and mortality. AF also poses a significant burden on healthcare and is associated with an annual estimated cost of 7 billion US dollars [2]. Yet, despite its high incidence and financial impact, AF management remains unsatisfactory. Traditional treatments to restore and maintain normal heart rate, namely, electrical cardioversion followed by initiation and lifelong maintenance with antiarrhythmic drugs [3], fail in most patients [4-6]. Catheter ablation is a rapidly emerging alternative. This curative approach seeks to suppress the sources of electrical dysynchrony by converting the cells responsible for the arrhythmia to inactive scar tissue. Radiofrequency (RF) energy is applied through a specialized catheter introduced via the venous system into the left atrium (LA) of the heart (Figure 1). This approach offers a true cure, obviating the need for lifelong medication. The success rate, however, is low at only 40-80%. At least two major obstacles to this treatment approach have been identified: (1) inability to distinguish which patients will (or will not) benefit from the procedure, and (2) inability to rapidly identify the extent of lesion ablation immediately post-procedure, as well as subsequent to scarification. In this regard, MRI offers the most promising technology to overcome these obstacles. Rapid, automated image processing and analysis have been identified as the rate-limiting steps to the development of practical MRI-based therapies.
Specific Aims
1. Develop and validate image-based longitudinal diagnostic indices for AF. We will (1) apply emerging registration technologies that use image and shape-based priors to study longitudinal changes in atrial morphology; (2) develop nonlinear registration approaches that do not require identification of common landmarks between image sets or between different imaging modalities; and (3) implement new methods to achieve robust multimodal and pre-/post-imaging registration in the face of uncompensated image distortion from respiration and cardiac motion and in the presence of local intensity changes due to treatment.
2. Develop automatic segmentation methods for the atrium and adjacent structures. Segmentation is the essential step for image-based analysis of patients with AF because it provides a quantitative index of the abnormal tissue substrate. Current segmentation software is largely manual and slow, limiting its utility to guide the ablation procedure. It also is technically simplistic, as it is based on setting intensity thresholds to differentiate normal from abnormal regions. To achieve this aim, we will integrate and implement more efficient and sophisticated 3D approaches into our workflow; help develop suitable priors; and establish effective parameters. These methods then will be validated against manual segmentation by experts. We anticipate that pre-ablation assessment of fibrosis, peri-ablation evaluation of lesions, and post-ablation visualization of scar formation each will require a different approach. Success in this aim will improve the efficiency of our own studies and provide tools for dissemination to our partner labs, thus enabling the first multicenter trial of MRI for AF management.
3. Develop an AF scoring scheme to evaluate disease progression and recovery from therapy. The NA-MIC Kit and Slicer 3 will be used to integrate, test, and provide feedback for all of the components of a fully integrated software environment that can provide segmentation, registration, and quantitative shape analysis capabilities for MRI-based management of AF. This workflow will incorporate other non-image information necessary to establish the scoring scheme. Such schemes exist for other forms of heart disease and are invaluable in setting standards for care, determining the appropriate time for intervention, and for screening and preventative medicine. The availability of a non-invasive scheme for monitoring disease progression will revolutionize the management of AF. To implement this scheme, we will require efficient algorithms, developed within the technical cores of NA-MIC, and an intuitive customizable user interface that gives open access to a database of previous results and population information. The system described would be new to our field and would fill a critical need.
Background
Tissue remodeling of the atrial wall is the hallmark of AF, a progressive disease that develops over time (months to years). Although AF may have a specific genetic component [7, 8], it is not a typical congenital disease. All hearts are capable of sustaining chronic AF in the presence of rapid stimulation. Hence, the expression “Atrial fibrillation begets atrial fibrillation” [9]. The mechanisms for this transformation are only partially known [9, 10]. However, the current focus on tissue remodeling and its putative role in AF makes the image-based approach ideal.
The Comprehensive Arrhythmia Research and MAnagement (CARMA) Center at the University of Utah is a world leader in the rapidly emerging field of MRI-managed evaluation and ablation of AF [11-15]. Other groups have begun to recognize the potential of this approach [16-18] and to investigate and validate some of our findings [19, 20]. Still others are developing new refinements of the MRI technique driven by the specific needs of this application domain [21-25].
Investigators
NAME | DEGREE | INSTITUTION | EXPERIENCE | ROLE |
ROB MACLEOD | PH.D. | UTAH | BIOENGINEERING | DBP PI |
JOSH CATES | PH.D. | UTAH | COMPUTER SCIENCE | RESEARCH ASSOCIATE |
ALLEN TANNENBAUM | PH.D. | GEORGIA TECH | COMPUTER SCIENCE | LEAD TECHNICAL CONTACT |
ROSS WHITAKER | PH.D. | UTAH | COMPUTER SCIENCE | LEAD TECHNICAL CONTACT |
JIM MILLER | PH.D. | GE GLOBAL | RESEARCH ENGINEERING | LEAD TECHNICAL CONTACT |
Methods
Preliminary investigation by CARMA has identified image processing and analysis as the rate-limiting step to the development of MRI-based therapies. Novel forms of MRI can be used to evaluate new patients, predict success before ablation [12-14], analyze outcomes post-ablation, and guide repeat ablations [11]. While the results have been promising [16-18], MRI-based therapies urgently require advanced tools and software to support efficient workflows and accelerate the quantification and analysis of images. Processing each MR image set from a new patient requires extensive segmentation of organs and regions within organs.
This process currently takes 2-3 hours of manual labor per case by experienced technicians. Moreover, the results, while quantitative, display large variance because of the subjective nature of critical thresholds. To effectively deliver catheter ablation, the post-ablation analysis (i.e., rapid registration of pre- and post-ablation images, segmentation of the atria, identification of tissue changes from ablation, and interactive visualization of the heart and ablated regions) must be accomplished within 30 minutes, while the patient is still on the procedure table and available for adjustments. Current practice, even in advanced labs, is constrained by the labor-intensive image analysis process. The lack of adequate image processing techniques hinders the interpretation of post-ablation imaging, often necessitating a full repeat procedure for recurrent AF at a later date, which increases costs and patient discomfort. An integrated customized set of automated software tools for image processing and analysis, based on state-of-art 3D image processing, would dramatically improve all stages of patient management. The NA-MIC Kit, Slicer, and supporting algorithms form the basis for such development.
A survival curve showing the rate of continued normal sinus rhythm as a function of time after ablation for patients grouped according to the level of late gadolinium enhancement (LGE) on MRI images acquired before ablation therapy is depicted in Figure 2. This curve shows promising findings from CARMA patients and illustrates the need for improvements in image analysis. These results suggest that late gadolinium enhancement (LGE), which is thought to be a measure of tissue fibrosis, is predictive of outcome in ablation. Patients with very low levels of LGE enhancement (< 5%) have 100% maintenance of normal rhythm while those with extensive enhancement (> 35%) have only about 10% success after one year. Although these extremes are useful for differentiating ideal patients from those with little chance of success, these two groups account for less than 10% of AF patients. We need to define enhancement thresholds that can predict risk for intermediate levels of enhancement. Current methods are not sufficiently accurate or robust for performing segmentations or identifying enhancement.
Improved algorithms for image processing and analysis are needed before radiofrequency ablation can be evaluated in all patients irrespective of the level of their disease.
Achieving these specific aims will require close, bidirectional interactions with the Algorithms team, a task greatly facilitated by our close physical proximity at Utah and by the choice of a highly experienced postdoctoral fellow to carry out the technical steps. Members of the Algorithms team already have received briefings on existing practices, explanations of the nature of the clinical needs, and have access to many examples of clinical MRI data from over 800 separate scans currently in the CARMA database. These interactions have produced
promising preliminary findings, including landmark-free registration of atrial geometry and statistical shape analysis of left atrial morphology across patient groups and longitudinally for individual patients as they recover after ablation therapy. An example of some of our preliminary results is shown in Figure 3.
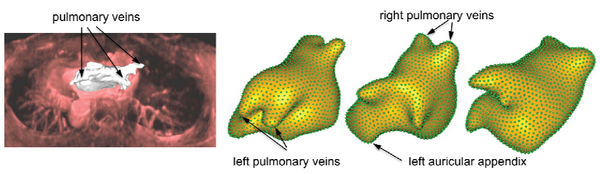
Clinicians in CARMA conduct over 20 ablations per week and see several times this number of patients in clinic, generating up to 50 separate MRI studies weekly, all of which require careful image processing and quantitative evaluation. All indicators suggest continued aggressive growth of this approach in our program and in many other clinics around the world, with CARMA at the center of a recently created multicenter clinical trial that will generate at least 50 additional scans per week. The urgent need to process and analyze such large volumes of real-world data in a timely manner will guarantee the widespread testing of tools developed within NA-MIC for this project.
The technical support for this DBP will be provided by Dr. Josh Cates, who recently received a PhD in Computer Science based on research in image processing and analysis. His mentor, Dr. Ross Whitaker, is PI of the Algorithms section of the Computer Science Core. Dr. Cates recently joined CARMA to assume leadership in the development, implementation, and validation of image analysis techniques and software. His expertise extends beyond algorithms to code development, as he was one of the original authors of ITK and is well acquainted with the software engineering practices and design ideas that also are shared within the NA-MIC Kit.
Dr. Rob MacLeod, a senior researcher in cardiac electrophysiology and biophysics, co-founder of CARMA, and associate director of SCI, is uniquely positioned to coordinate and direct this DBP.
Connection to Cores and other DBPs
This DBP will rely heavily on the Computer Science Core to identify specifically appropriate technical approaches and provide support for implementation and testing of new methods and workflows. The development efforts on pre- and post-therapy image registration, efficient segmentation of anatomical objects, including longitudinal processing, and work towards a patient scoring system for improved decision-making, will be shared with similar efforts of the other DBPs.
Deliverable, Timeline, and Impact
SPECIFIC AIMS | Year 1 | Year 2 | Year 3 |
Aim 1 | Evaluate existing algorithms | Integrate linear and nonlinear registration into prototype workflow | Optimize tools, tests, and validation documentation |
Aim 2 | Evaluate existing and implement new tools for atrial wall segmentation and for tissue characterization | Joint segmentation of pre- and post-treatment data, efficient implementations through software and hardware acceleration | Refine segmentation tools, tests and validation, integrate post-treatment image segmentation into clinical workflow, documentation |
Aim 3 | Design of segmentation and registration workflow and application-specific GUI | Prototype workflow system for integrated registration and segmentation, pre-/post-analysis and visualization. Tests on existing database. | Tests on image data shared with other labs; establish database also with nonimage information for prototypical scoring system, training, and dissemination. |
Utah investigators will interact directly with NA-MIC PIs to develop new and refine existing algorithms for MRI-based registration and segmentation techniques to treat and manage AF. DBP activities at Utah will occur in consultation with the Lead NA-MIC Technical Contacts for Algorithms (Whitaker, Tannenbaum) and Engineering (Miller). Once completed and rigorously validated, these workflows will (1) be applied to the AF patient data described above, (2) made available for open dissemination via the NA-MIC website, and (3) form the basis for training and educational materials for NA-MIC investigators and the AF community. Results will be featured in presentations at scientific conferences, organized training events and workshops as a way to disseminate tool capabilities. Where possible, tutorials on how to use the NA-MIC technology for other AF-related projects will be made available. Finally, the DBP-PI will attend each NA-MIC All-Hands-Meeting to discuss the DBP with NA-MIC PIs, and report on new developments and progress. NA-MIC will benefit from this DBP by exposure to a rapidly growing clinical community involved with diagnosis, treatment, and management of AF patients. Even beyond AF, this DBP collaboration and associated clinical procedures and research, reaches a much wider clinical community involved with personalized medicine, which, given novel image processing tools, could better integrate newly emerging imaging into the current clinical workflow.
References
- Benjamin EJ, Levy D, Vaziri SM, D’Agostino RB, Belanger AJ, Wolf PA. Independent risk factors for atrial fibrillation in a population-based cohort. The Framingham Heart Study. Jama. 1994;271(11):840-4. PMID: 8114238.
- Coyne KS, Paramore C, Grandy S, Mercader M, Reynolds M, Zimetbaum P. Assessing the direct costs of treating nonvalvular atrial fibrillation in the United States. Value Health. 2006;9(5):348-56. PMID: 16961553.
- Falk RH. Atrial fibrillation. N Engl J Med. 2001;344(14):1067-78. PMID: 11287978.
- Brodsky MA, Allen BJ, Walker CJ, 3rd, Casey TP, Luckett CR, Henry WL. Amiodarone for maintenance of sinus rhythm after conversion of atrial fibrillation in the setting of a dilated left atrium. Am J Cardiol. 1987;60(7):572-5. PMID: 3630939.
- Crijns HJ, Van Gelder IC, Van der Woude HJ, Grandjean JG, Tieleman RG, Brugemann J, et al. Efficacy of serial electrical cardioversion therapy in patients with chronic atrial fibrillation after valve replacement and implications for surgery to cure atrial fibrillation. Am J Cardiol. 1996;78(10):1140-4. PMID: 8914878.
- Van Gelder IC, Crijns HJ, Tieleman RG, Brugemann J, De Kam PJ, Gosselink AT, et al. Chronic atrial fibrillation. Success of serial cardioversion therapy and safety of oral anticoagulation. Arch Intern Med. 1996;156(22):2585-92. PMID: 8951302.
- Christophersen IE, Ravn LS, Budtz-Joergensen E, Skytthe A, Haunsoe S, Svendsen JH, et al. Familial aggregation of atrial fibrillation: a study in Danish twins. Circ Arrhythm Electrophysiol. 2009;2(4):378-83.
- Watanabe H, Darbar D, Kaiser DW, Jiramongkolchai K, Chopra S, Donahue BS, et al. Mutations in sodium channel beta1- and beta2-subunits associated with atrial fibrillation. Circ Arrhythm Electrophysiol. 2009;2(3):268-75.
- Wijffels MC, Kirchhof CJ, Dorland R, Allessie MA. Atrial fibrillation begets atrial fibrillation. A study in awake chronically instrumented goats. Circulation. 1995;92(7):1954-68. PMID: 7671380.
- Allessie MA, Boyden PA, Camm AJ, Kleber AG, Lab MJ, Legato MJ, et al. Pathophysiology and prevention of atrial fibrillation. Circulation. 2001;103(5):769-77. PMID: 11156892.
- McGann CJ, Kholmovski EG, Oakes RS, Blauer JJ, Daccarett M, Segerson N, et al. New magnetic resonance imaging-based method for defining the extent of left atrial wall injury after the ablation of atrial fibrillation. J Am Coll Cardiol. 2008;52(15):1263-71. PMID: 18926331.
- Badger TJ, Adjei-Poku YA, Marrouche NF. MRI in cardiac electrophysiology: the emerging role of delayed-enhancement MRI in atrial fibrillation ablation. Future Cardiol. 2009;5(1):63-70. PMID: 19371204.
- Badger TJ, Oakes RS, Daccarett M, Burgon NS, Akoum N, Fish EN, et al. Temporal left atrial lesion formation after ablation of atrial fibrillation. Heart Rhythm. 2009;6(2):161-8.
- Oakes RS, Badger TJ, Kholmovski EG, Akoum N, Burgon NS, Fish EN, et al. Detection and quantification of left atrial structural remodeling with delayed-enhancement magnetic resonance imaging in patients with atrial fibrillation. Circulation. 2009;119(13):1758-67. PMID: 19307477.
- Akoum N, Marrouche NF. Real-time imaging in electrophysiology: from intra-cardiac echo to real-time magnetic resonance imaging. Europace. 2009;11(5):539-40. PMID: 19359332.
- Hauser TH, Peters DC, Wylie JV, Manning WJ. Evaluating the left atrium by magnetic resonance imaging. Europace. 2008;10 (Suppl 3):iii22-iii7.
- Kolandaivelu A, Lardo AC, Halperin HR. Cardiovascular magnetic resonance guided electrophysiology studies. J Cardiovasc Magn Reson. 2009;11(1):21. PMID: 19580654.
- Saremi F, Tafti M. The role of computed tomography and magnetic resonance imaging in ablation procedures for treatment of atrial fibrillation. Semin Ultrasound CT MR. 2009;30(2):125-56. PMID: 19358443.
- Peters DC, Wylie JV, Hauser TH, Nezafat R, Han Y, Woo JJ, et al. Recurrence of atrial fibrillation correlates with the extent of post-procedural late gadolinium enhancement: a pilot study. JACC Cardiovasc Imaging. 2009;2(3):308-16. PMID: 19356576.
- Wylie JV, Jr., Peters DC, Essebag V, Manning WJ, Josephson ME, Hauser TH. Left atrial function and scar after catheter ablation of atrial fibrillation. Heart Rhythm. 2008;5(5):656-62. PMID: 18452866.
- Krishnam MS, Tomasian A, Malik S, Singhal A, Sassani A, Laub G, et al. Three-dimensional imaging of pulmonary veins by a novel steady-state free-precession magnetic resonance angiography technique without the use of intravenous contrast agent: initial experience. Invest Radiol. 2009;44(8):447-53. PMID: 19561516.
- Chyou JY, Biviano A, Magno P, Garan H, Einstein AJ. Applications of computed tomography and magnetic resonance imaging in percutaneous ablation therapy for atrial fibrillation. J Interv Card Electrophysiol. 2009;26(1):47-57. PMID: 19521756.
- Rossillo A, Indiani S, Bonso A, Themistoclakis S, Corrado A, Raviele A. Novel ICE-guided registration strategy for integration of electroanatomical mapping with three-dimensional CT/MR images to guide catheter ablation of atrial fibrillation. J Cardiovasc Electrophysiol. 2009;20(4):374-8. PMID: 19017352.
- Tops LF, Schalij MJ, den Uijl DW, Abraham TP, Calkins H, Bax JJ. Image integration in catheter ablation of atrial fibrillation. Europace. 2008;10 (Suppl 3):iii48-iii56.
- Sra J, Ratnakumar S. Cardiac image registration of the left atrium and pulmonary veins. Heart Rhythm. 2008;5(4):609-17. PMID: 18325847.